
Video: Flying gait: what happens to the protein inside a living cell
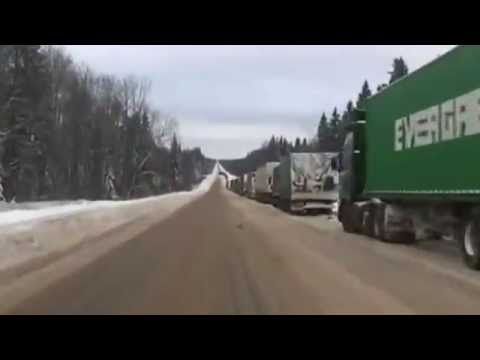
2024 Author: Seth Attwood | [email protected]. Last modified: 2023-12-16 15:55
Many do not even suspect how truly amazing processes are taking place inside us. I suggest you look further at the microscopic world, which you managed to see only with the advent of the latest new generation electron microscopes.
Back in 2007, Japanese researchers were able to observe under a microscope the work of one of the "molecular motors" of a living cell - the walking protein myosin V, which can actively move along the actin fibers and drag the weights attached to it. Each step of myosin V begins with the fact that one of its "legs" (back) is separated from the actin filament. Then the second leg bends forward, and the first freely rotates on the "hinge" connecting the legs of the molecule, until it accidentally touches the actin filament. The end result of the chaotic movement of the first leg turns out to be strictly determined due to the fixed position of the second.
Let's find out more about this …
… kinesin walks like this

Any active movements performed by living organisms (from the movement of chromosomes during cell division to muscle contractions) are based on the work of "molecular motors" - protein complexes, parts of which are able to move relative to each other. In higher organisms, the most important of the molecular motors are myosin molecules of various types (I, II, III, etc., up to XVII), which are able to actively move along the actin fibers.
Many "molecular motors", including myosin V, use the principle of walking motion. They move in discrete steps of approximately the same length, and alternately one or the other of the two "legs" of the molecule is in front. However, many details of this process remain unclear.
Researchers at the Department of Physics, Waseda University in Tokyo have developed a technique that allows you to observe the work of myosin V in real time under a microscope. To do this, they constructed a modified myosin V, in which the leg shafts have the property of firmly "sticking" to tubulin microtubules.
By adding fragments of microtubules to the solution of modified myosin V, the scientists obtained several complexes in which a piece of a microtubule adhered only to one leg of myosin V, while the other remained free. These complexes retained the ability to "walk" along the actin fibers, and their movements could be observed, since the fragments of microtubules are much larger than myosin itself, and, moreover, they were labeled with fluorescent labels. In this case, two experimental designs were used: in one case, an actin fiber was fixed in space, and the observations were carried out over the movement of a microtubule fragment, and in the second, a microtubule was fixed and the movement of an actin fiber fragment was observed.

As a result, the “gait” of myosin V was studied in great detail (see the first figure). Each step begins with the “back” leg of myosin separating from the actin fiber. Then that leg, which remains attached to the fiber, leans forward sharply. It is at this moment that energy is consumed (ATP hydrolysis occurs). After that, the “free” leg (green in the figures) begins to dangle chaotically on the hinge. This is nothing more than Brownian motion. At the same time, by the way, scientists were able to show for the first time that the hinge connecting the legs of myosin V does not restrict their movements at all. Sooner or later, the green leg touches the end of the actin filament and attaches itself to it. The place where it will attach to the string (and therefore the stride length) is entirely determined by the fixed inclination of the blue leg.
In the experiment, the search for the actin filament with the free leg of myosin V took several seconds; in a living cell, this apparently occurs faster, since there myosin walks without weights on its legs. Weights - for example, intracellular vesicles surrounded by membranes - are attached not to the legs, but to that part of the molecule, which is depicted as a "tail" in the figure.
Recommended:
The solar system is a living cell of the universe

As direct participants in the milestone events in the history of mankind, we all together and each separately will have to voluntarily or forcibly make our own existential
What happens if you remove half of the brain?

The human brain is the command center of the nervous system. It receives signals from the senses and transmits information to the muscles, and in certain areas of the left or right hemisphere, depending on the activity, forms new neural connections, in other words, it learns. But what if, as a result of treatment, a person physically removed one of the hemispheres?
What happens with different ppm blood alcohol levels?

What exactly happens to the body with different ppm of alcohol in the blood, and how does this affect us? We will deal with Professor Janne Tolstrupa, head of research at the Danish State Institute of Health, including studying the relationship between alcohol and health
What happens to the city after 25 years of being under water

In the 1920s, a resort town was built on the shores of Lake Epecuen in Argentina. The unique healing properties of the reservoir attracted thousands of people from all over the world who wanted to improve their health and relax on its shores. The resort thrived for over half a century until it sank underwater in 1985
Nuclear reactor in a living cell

What Vladimir Vysotsky, Doctor of Physics and Mathematics, Professor, Head of the Department of the KNU named after TG Shevchenko, does not fit into the usual scientific framework. His experiments recorded that biological systems can, relatively speaking, arrange small nuclear reactors inside themselves